From how to capture CO2 in the atmosphere to how to deliver lifesaving drugs, scientists can answer a variety of questions by studying the movement of nanoparticles.
Early last month, University researchers published a study describing how nanoparticles move and disperse in flowing fluids. Assistant Professor of Engineering Daniel Harris was the principal investigator and worked with researchers Stuart Thomson, Ajay Harishankar Kumar ScM’20 and Professor of Engineering and Physics Thomas Powers.
“Historically, (our research) goes back to the work of mathematician G. I. Taylor,” said Thomson, a postdoc in Harris’s lab. Taylor’s work described how shear flow, which is a type of fluid flow induced by a pressure gradient, causes spherical nanoparticles to disperse and spread out — an effect known as Taylor Dispersion. This effect is very important in fluid dynamics, Thomson said.
“It’s easier to do analysis and calculations on spherical particles, but in reality not everything is spherical,” said Kumar, who was the first author of the study. “In our study, we consider particles like an ellipsoid or (a) long-shaped rod. We wanted to see what kind of effect the shape would have on the properties” of the particles in the fluid, Kumar added.
To study the motion of ellipsoid-shaped particles, which are also referred to as elongated rods, the researchers used a method known as a Monte Carlo simulation — a type of computational algorithm that uses repeated random sampling. The researchers used the Oscar supercomputer from Brown’s Center for Computation and Visualization to run numerical simulations on nearly a million elongated particles subjected to shear fluid flow, Kumar said.
The Monte Carlo simulations considered both the fluid flow’s effect on the elongated rods as well as Brownian motion, or the random movement of particles in stagnant fluid, Thomson said. “There are some differential equations you can model (particle movement) with,” he added.
After running the simulations, the researchers analyzed the speed of the particles. They also described the spreading of the particles, which they used to calculate a dispersion coefficient, Kumar said.
“We actually found that rod-shaped particles spread much more than the spherical particles in high fluid flow,” Kumar said. The speed of the elongated rods seemed to decrease, but was statistically insignificant when compared to the increase in dispersion coefficient, he added.
Using the information from the simulations, the researchers were able to calculate a correction factor. “For real life applications … we can put a small correction factor to the spherical case and you get the rod-shaped case,” Kumar said.
The simulation method and theoretical model from this study can provide a useful modeling reference for other researchers in this field, wrote Yun Chen, a postdoctoral research associate at the University of Minnesota, in an email to The Herald. “If we are studying similar systems of particles … with different geometry of the particles, we could refer to this paper and modify their model … to be applied to our own system,” added Chen, who was not involved in the study.
The findings about the mean speed and dispersion rate of the rods can have applications in predicting the behaviors of non-spherical biological objects, such as bacteria and microscopic health technology devices, he added.
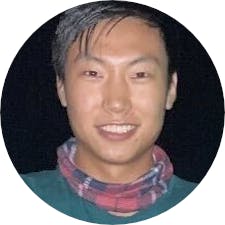
Jared is a Senior Staff Writer for Science and Research. He is a senior from Albuquerque, New Mexico studying physiology and biotechnology. Outside of The Herald he likes to fish, ride bikes and research the role of metals in human health and disease.