Researchers from multiple academic institutions collaborated on a study published last month, in which they imaged and investigated systems of active liquid crystals in three dimensions and investigated their movements and patterns. Several University professors were at the forefront of the theoretical work behind the study, which appeared on the cover of Science, a renowned research journal.
The results of the study offer new insights into a variety of fields, from medicine to computer technology.
In conventional nematic liquid crystals, tiny parallel rod-like particles align in near perfect order. In active nematics, however, each particle self-propels into continuous motion, interacting with surrounding particles to create intricate loops and patterns, said Professor Zvonimir Dogic at the University of California at Santa Barbara, who led the experimental efforts to model active nematics in 3D. Dogic is the co-principal investigator of the study alongside Assistant Professor Daniel Beller at the University of California at Merced and former postdoctoral research associate at Brown.
These disruptions in order are known as topological defects and are fundamental properties of nematic liquid crystals, Dogic said. Although scientists have a thorough understanding of these properties in two dimensions, they had not previously experimented with and imaged the crystals’ behaviour in three-dimensional contexts, he added.
But in this study, the researchers “could track and study topological defects” in 3D, which “was very special,” said Professor of Physics Robert Pelcovits, a theoretical physicist and a co-author of the study.
In two-dimensions, defects always appear as a pair with opposite charges, which ultimately cancel out and neutralize each other. Once the pair of charges is created, they separate and move away, the researchers said.
“In 3D, we’ve actually shown that the dynamics (are) very different,” Dogic said. The researchers discovered that the most common topological defects that occur in this environment are loops. Since the net charge of the particles is zero, “we get these loops that spontaneously appear out of nothing,” he added.
Unlike in 2D, particles in 3D defects also stay connected by disclination loops, which are like “a rubber band that holds (the particles) together,” Dogic said.
These experimental results drove theoretical work that sought to further explain the nature of these disruptions in order; it was led by Beller and University professors Pelcovits and Thomas Powers, professor of engineering and physics, according to a University press release. Theoreticians assembled sets of equations describing topological defects and compared simulated results to experimental ones.
According to Powers, another co-author of the study, the researchers discovered simple and universal patterns in 3D topological defects.
As it turns out, “only one kind of dynamical pattern dominates both experiments and theory,” said Dogic, “so that was very gratifying.”
“It was not easy to go from 2D to 3D,” Dogic added. In 2D, scientists can simply use a microscope to focus on a single plane, but expanding into 3D presented major technical challenges. To capture topological defects, researchers applied a complex technology called light sheet microscopy, as well as created a new artificial nematic material, in order to visualize the dynamics of an active system in 3D.
“By combining this technology and new biological materials,” said Dogic, “we were able to really visualize what’s happening in the samples with molecular resolutions” for extended periods of time with “fast temporal resolution.”
This breakthrough into 3D active systems can potentially have a wide-ranging impact. Professor Cristina Marchetti of the University of California at Santa Barbara, who studies active matter in relation to biology but was not involved in the study, said, “From a biological point of view, the flow that you see in this active system very much resembles flows you see in the cytoplasm, the fluid inside cells.”
Through understanding how to control movements in active systems, this research can be applied to make biomimetic devices, which artificially mimic biological systems, Pelcovits said.
Understanding topological defects is also key in the ever-growing technological industry, where nematic liquid crystals “are used to make all kinds of displays we use everyday, like our phones and our computer screens,” Marchetti said.
University researchers and Marchetti acknowledge that the current discoveries are still far from application, and there are many directions for future investigation. Researchers hope to zoom in further by isolating single 3D loops from the rest of their environments, adding externally driven movements and studying the effect of putting objects in active fluid, the researchers said.
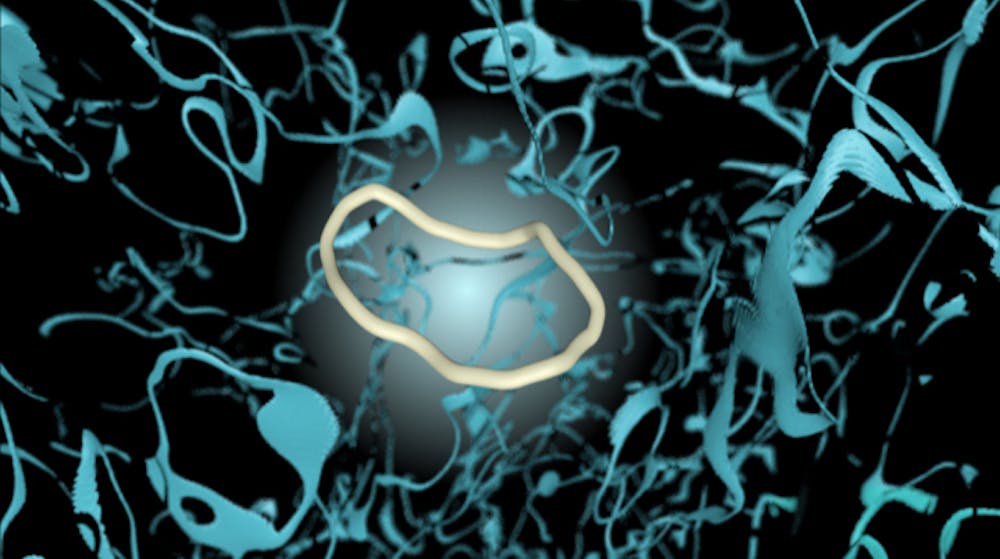
ADVERTISEMENT
More